TL;DR (too long; didn’t read)
The mountain Ofenkaulberg in North Rhine-Westphalia is made up of trachyte tuff. The rock formed as a result of volcanic eruptions that took place approximately 25 million years ago. These eruptions were the beginning of long lasting volcanic activities in the Siebengebirge. Tectonic activity in the Lower Rhine embayment is associated with the volcanic activity.
The mountain is characterised by a system of galleries which are evidence for a long and intense mining history. Furthermore, these tunnels were used during WW2 for weapon production. Nowadays all galleries are closed off for safety reasons. Modern technology has allowed a 3D model to be created and the tunnels are now accessible to the public in this safer way. The Siebengebirgsmuseum in Königswinter offers insightful information concerning the mountain and its history.
Introduction
The elongated, 250 m high Ofenkaulberg is located in the central part of the Siebengebirge, close to the city of Königswinter in North Rhine-Westphalia (Fig. 1). The Ofenkaulberg is not as famous as the neighbouring Petersberg or Drachenfels, but it is also significant. The secrets of the mountain are known only by the locals, as it is located within a nature reserve and hence not accessible to the public. Some hiking trails pass by in the vicinity. The distinctive features of the mountain are mining galleries – the so called ‘Ofenkaulen’. The galleries are the result of hundreds of years of trachyte tuff mining. These stones were preliminary used for the construction of ovens; hence the German name Ofenkaulen which literally translates to “ovenmine”. The mining industry was economically important for the city of Königswinter until the 1920s. The galleries were used during WW2 as a place for weapon construction. Labourers were forced to work underground. The local population used the mines as shelter during the last weeks of the war. Nowadays the Ofenkaulen are home to eight different species of bats.
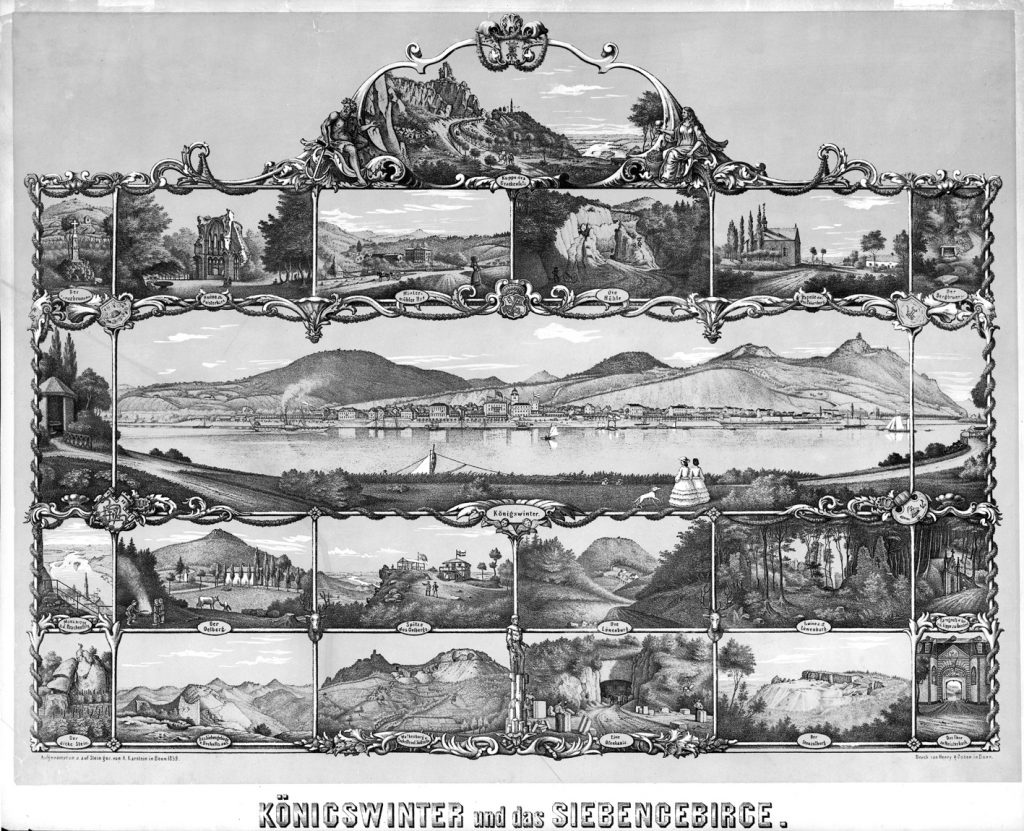
The history of the Ofenkaulen was investigated in a joint interdisciplinary project involving the state authority, the Siebengebirgsmusem, the city of Königswinter and the Biologische Station Rhein-Sieg-Kreis e. V. The geological survey NRW, the LVR-Amt für Bodendenkmalpflege and the Bonner Arbeitsgemeinschaft für Fledermausschutz (BAFF) were also involved. The results of this project are summarised in the book „Zeugen der Landschaftsgeschichte im Siebengebirge. Teil 2: Der Ofenkaulberg, Petersberg 2020“ and are the subject of a special exhibition at the Siebengebirgsmuseum.
Geology
The Siebengebirge is located south of the city of Bonn at the transition of the edge of the Lower Rhine Embayment and the Rhenish Slate Massif (Fig. 2). Extensional tectonics led to the subsidence of the Lower Rhine Embayment some 25 million years ago when the North Sea transgressed into the area. Volcanic activity started simultaneously and the Siebengebirge was formed (Fig. 3). The Ofenkaulberg is part of this volcanic area (Fig. 4).
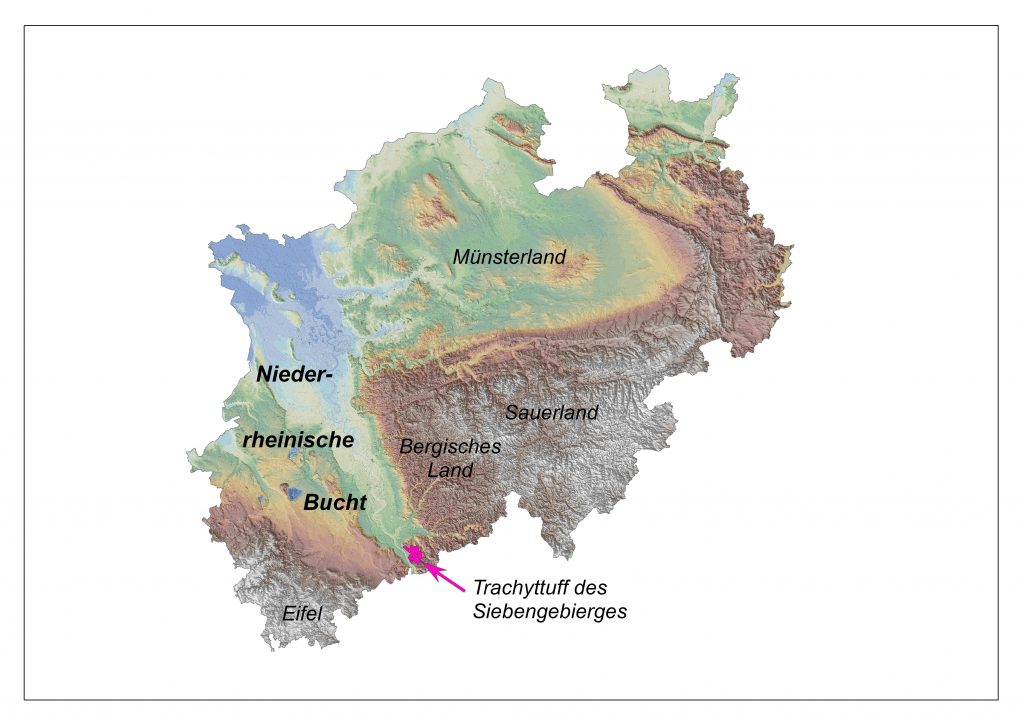
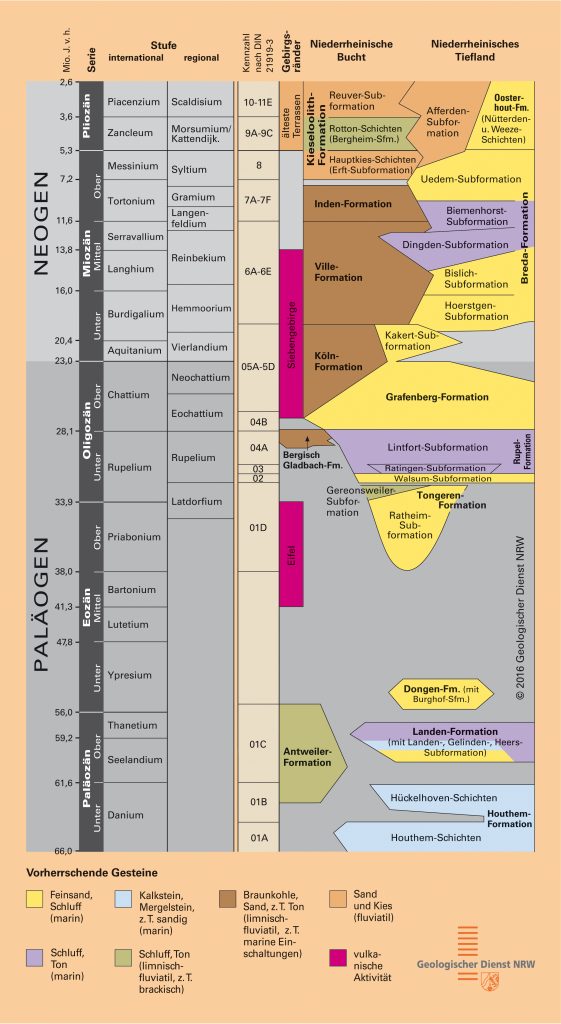
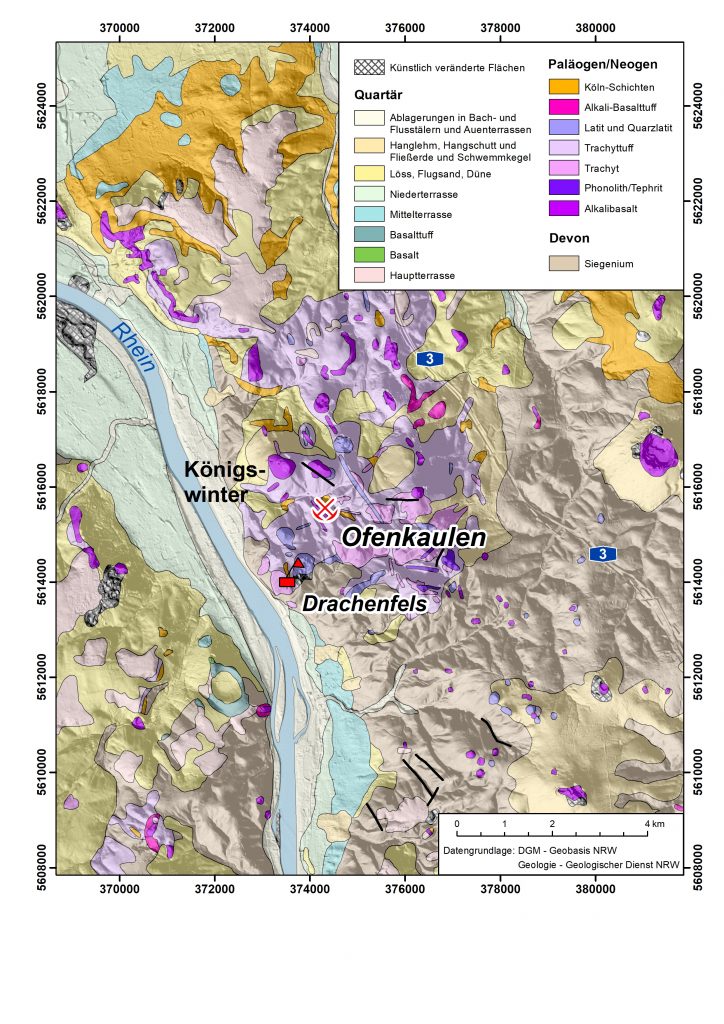
The volcanic activity in the Siebengebirge started with an explosive phase. An area of approximately 100 km2 was covered with 200 m thick deposits of well-layered light trachyte tuff, consisting of ash and pumice. Volcanic rocks of basaltic and trachytic composition intruded into the sequence shortly after this first phase. Some of these intrusions did not penetrate the surface (e.g. Drachenfels). Folded lower Devonian clay and siltstones make up the base of the volcanic rocks. Cross sections in figure 5 and 6 illustrate the situation. Dating evidence suggests that the volcanic activity lasted for an approximate period of 18 to 25,5 million years.
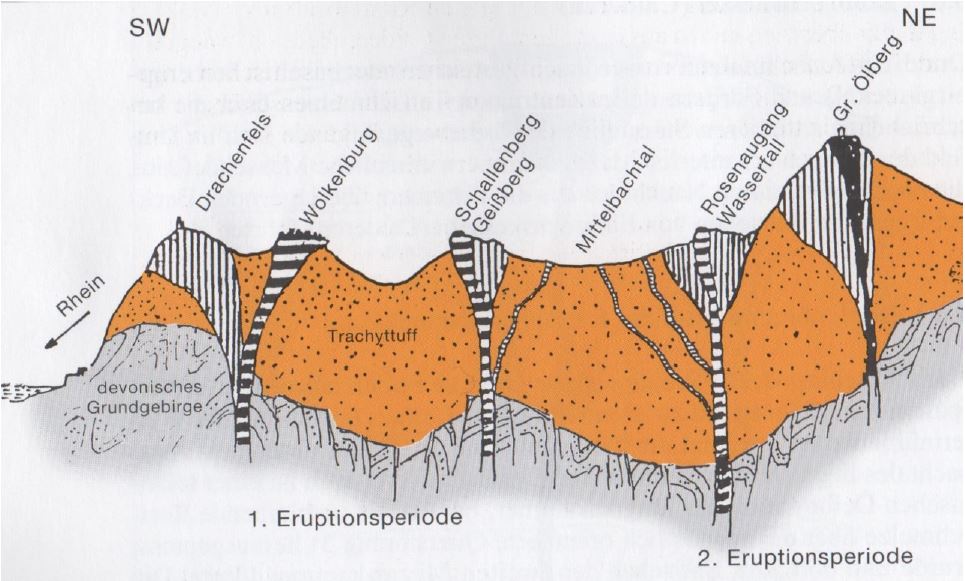
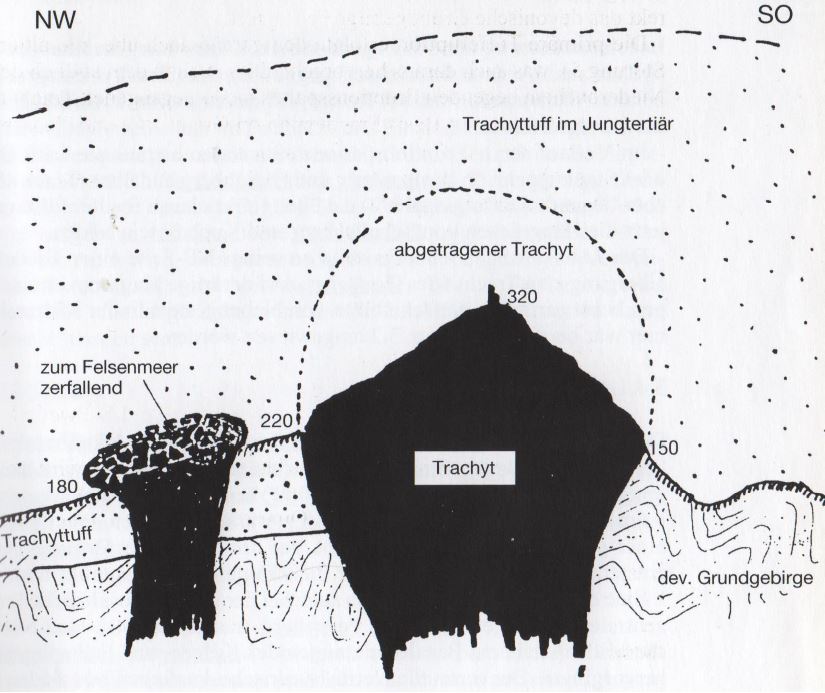
The landscape today is the result of Neogene and Quaternary erosion which affected the weakly cemented tuff deposits. The volcanic rocks are more resistant and form steep hills (Frechen, 1976). The remaining thickness of the trachyte tuff is approximately 20 m. In the central part of the Siebengebirge, the thickness is still 200 m. As a result of the uplift of the Rhenish Slate Massif, the Rhine river has cut deeply into the rock sequence.
The trachyte tuff is rather the product of repeated explosive volcanic activity than pyroclastic activity. The sequence indicates that the airborne volcanic products were deposited in a lake. Subaquatic debris flows led to the redistribution of the material (Moll, 2006). An interesting fact is that the pumice first floated on the lake surface before the pore space filled with water and the pumice sank to the bottom. Further sedimentological evidence for subaquatic deposition are cross bedding, graded bedding and dewatering structures.
The layered trachyte tuff is well exposed in the Ofenkaulen and has a thickness of 37 m here. Thin, fine grained layers intercalate with coarser ones. Numerous pumice stones of various sizes are characteristic of the trachyte tuff of the Ofenkaulen. Cross bedding and graded bedding are evident (Fig. 7 and 8). The layers dip by < 10° towards the north. Basaltic dykes within the tuff are exposed within the mine. They follow NW-SE striking faults of the Lower Rhine Embayment.
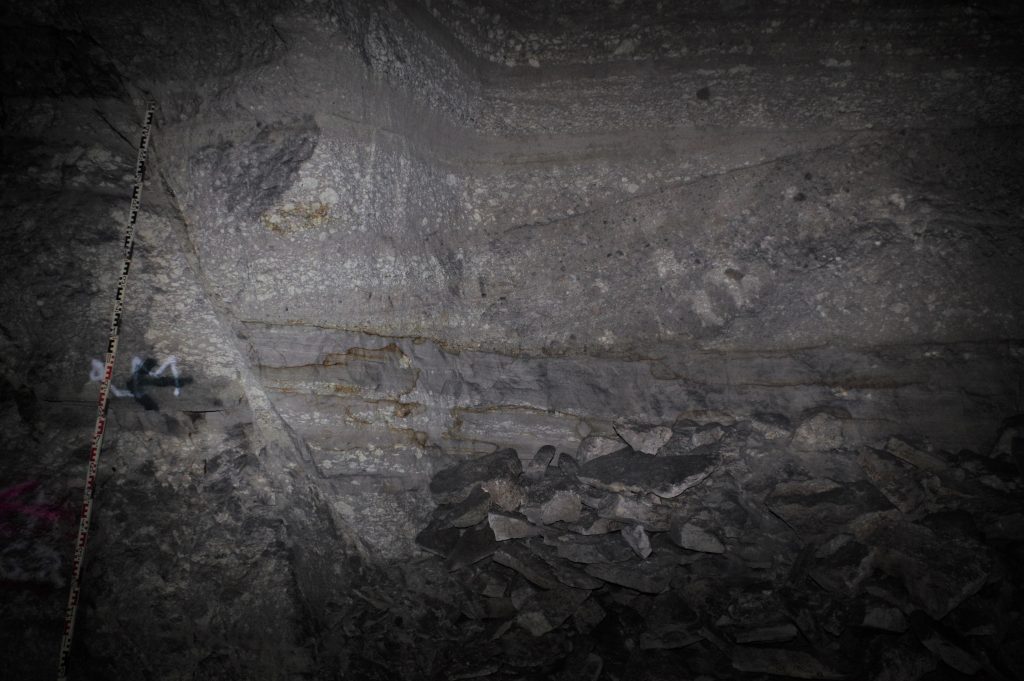
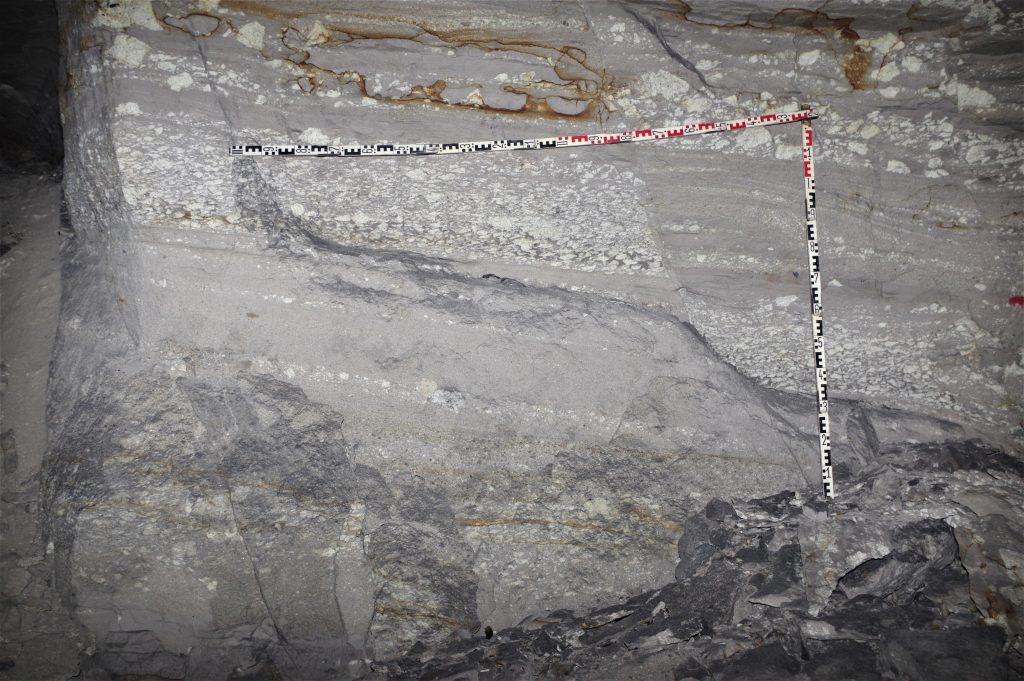
Mining history
While the quarrying of trachyte, latite and basalt left large scars on the landscape of the Siebengebirge, the predominantly underground mining of trachyte tuffs at the Ofenkaulberg remained mostly hidden.
Most probably from the late Middle Ages (1397), but certainly from 1635, trachyte tuff was extracted from the so-called Ofenkaulen (Kaulen = pits) for the baking oven construction that gave them their name. The fire-resistant stone is relatively easy to quarry, has good thermal properties and can be processed into large oven slabs due to its homogeneity. The tuff gained only limited importance as a building stone for vaults or other interior walls because it is not weather-resistant and needs to be protected from frost. It was also used for feed and water troughs and as a stove pipe socket.
From the Middle Ages until around 1700, stone was quarried from the surface (Fig. 7) – a phase that can only be traced today through the structure of the terrain, for example, through heavily decayed scarp slopes. The beginning of underground mining can also not be precisely determined. At the end of the 18th century, however, geological reports and travelogues already contain descriptions of the enormous dimensions of underground mining with halls up to 10 m in height. From the beginning of the 19th century, mining plans reveal a phase of complete exploitation of the middle level between 1826 and 1859, which led to the construction of new pits from 1860 to 1880. In the following peak phase of mining between 1880 and 1914, numerous shafts also opened up deeper levels (Fig. 10). This growth was interrupted by the First World War, which hit small businesses particularly hard as men were called up for military service. As a result, the oven-building trade also lost touch with modern oven-building technology and consequently more and more quarries were abandoned. After a Cologne armaments company moved parts of its production to the underground mining galleries during the Second World War (Fig. 11), the last remaining Königswinter oven builder finally also ceased his quarrying activities in 1957.
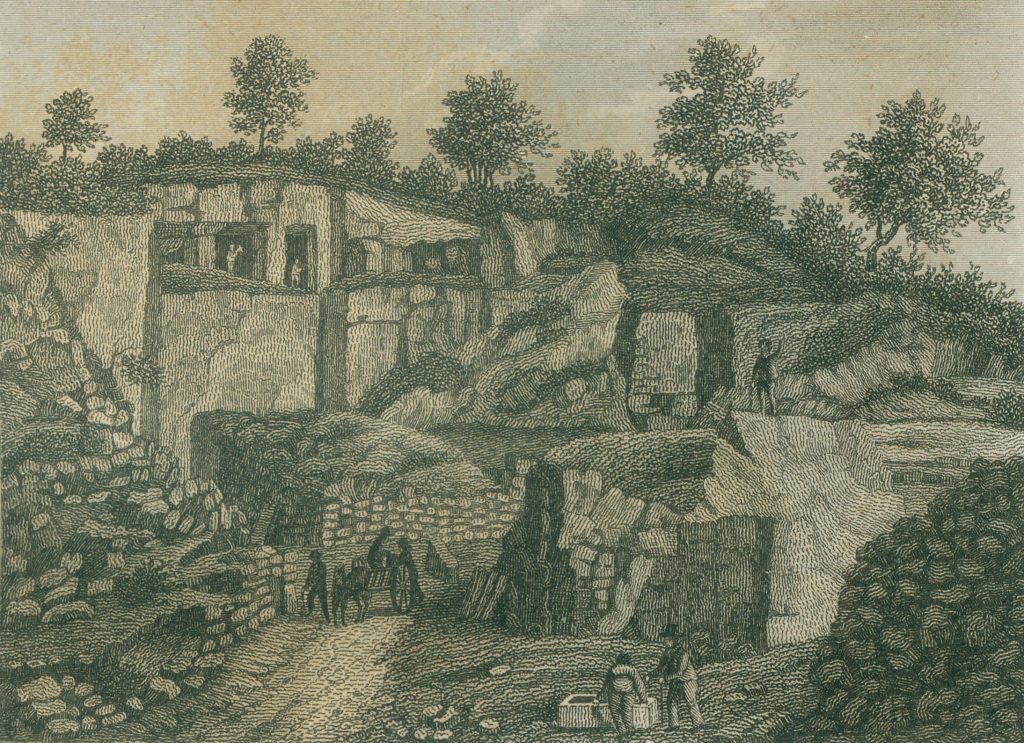
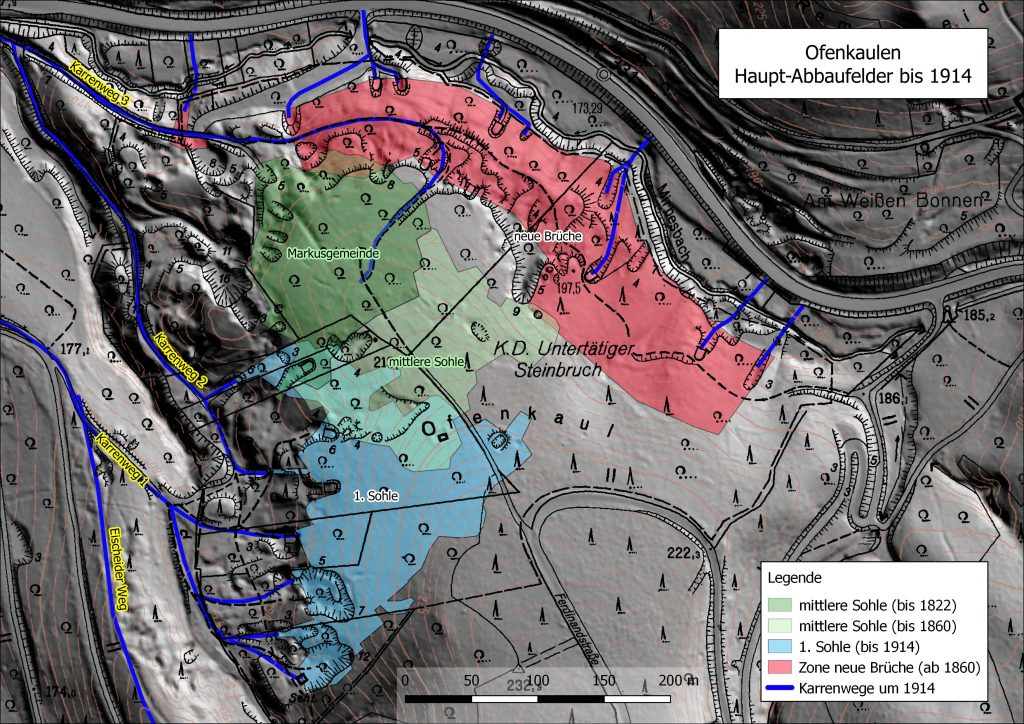
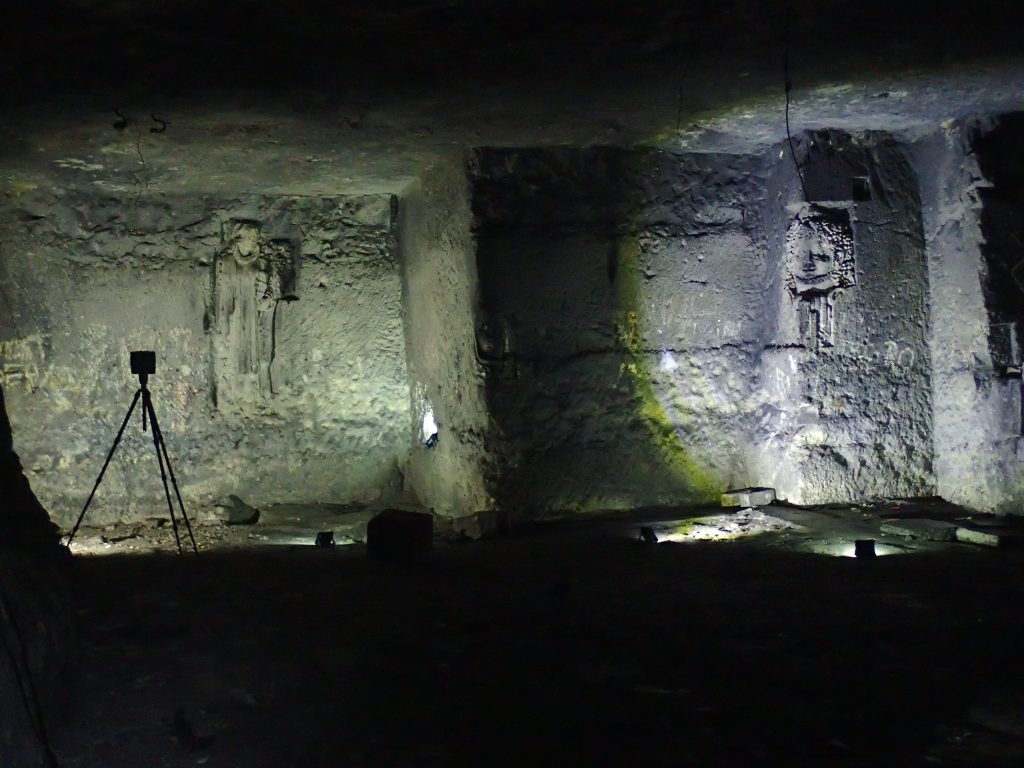
At the peak of quarrying, the Ofenkaulberg was divided into a large number of land parcels, on which up to 24 small companies (1910) with a total of about 50 men quarried stone. Initial calculations suggest that over the centuries at least 50,000 m2 of rock was extracted underground, whereby the various small parcel ownership and mining rights led to an extensive, labyrinthine system of tunnels. In many cases, no attention was paid to the stability of the mining works, which means that today there is a high risk of these structures collapsing, making them extremely dangerous (Fig. 12).
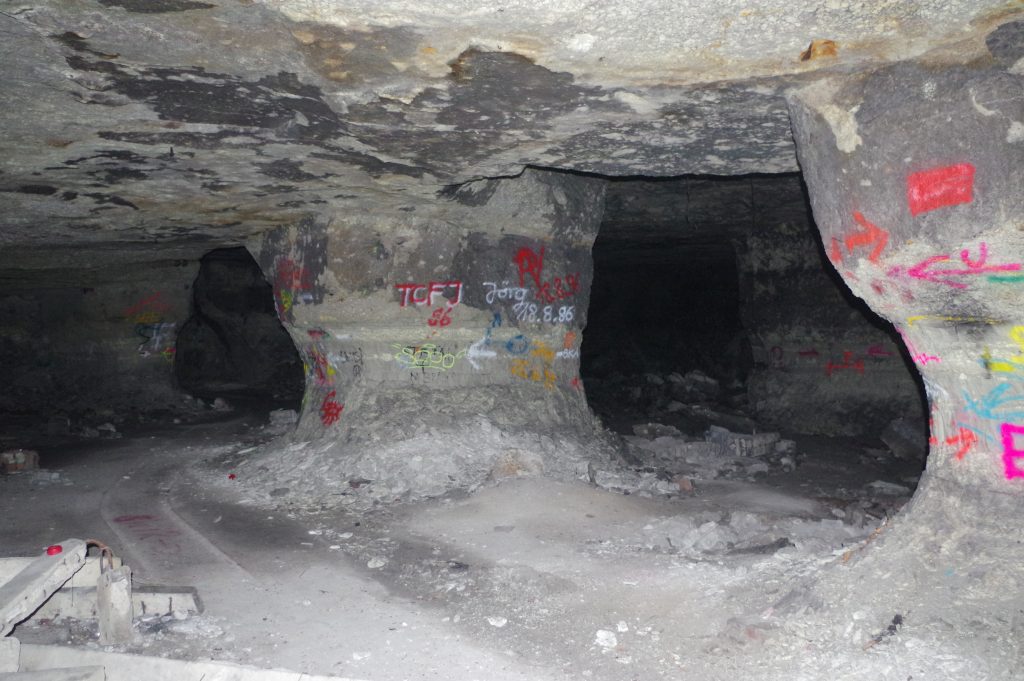
Methods
A number of methods have been established in recent years for the three-dimensional digital recording of geological objects. In the case of the geosites described so far in the 30 Geotope³ project, the focus has been on drone-based photogrammetry. In this process, a large number of photos are computed into a 3D model. For the present model, a terrestrial laser scanner (TLS) was used (Faro Focus 3DX330). A total of 157 device locations or individual scans were required to record the first floor as well as the two air shafts and the passageways to the lower floor levels. The data was processed with the software FaroScene and the open source freeware CloudCompare.
With the help of laser beams and the measured phase difference between the transmitted and received laser signal, the distance to the object is determined very precisely. With modern laser scanners, an object can be scanned or measured with millions of such measurements in a few minutes and displayed as a three-dimensional digital point cloud with point distances of a few millimetres. The laser scanner operates in the near-infrared spectrum with a wavelength of 1550 nm, which is invisible and harmless to the human eye. The great advantage of this method compared to photogrammetry is the fact that it is an active measuring method independent of a separate light source and thus ideal for underground surveys.
Various sensors integrated in the scanner, such as GPS receiver, compass, barometric altimeter and inclinometer normally ensure precise positioning in the geographic coordinate system. In enclosed spaces or underground, such as the furnace caverns, the location initially refers to a local coordinate system.
In addition to the three-dimensional visualisation of an outcrop, the virtual 3D point clouds serve as a basis for a variety of other geoscientific and geotechnical evaluations. The position and dimensions or even the volume of the scanned objects can be determined exactly. Another important aspect is the characterisation of geological interfaces or structures, such as the orientation of fractures and faults as well as the bedding of the rock layers. By blending the laser scan data with the digital elevation model, the positional relationships of the mine structure to the ground surface become clear (Fig. 13). The thickness of the overlying rock and the free span of the underground galleries were determined in order to assess the stability of the structure.
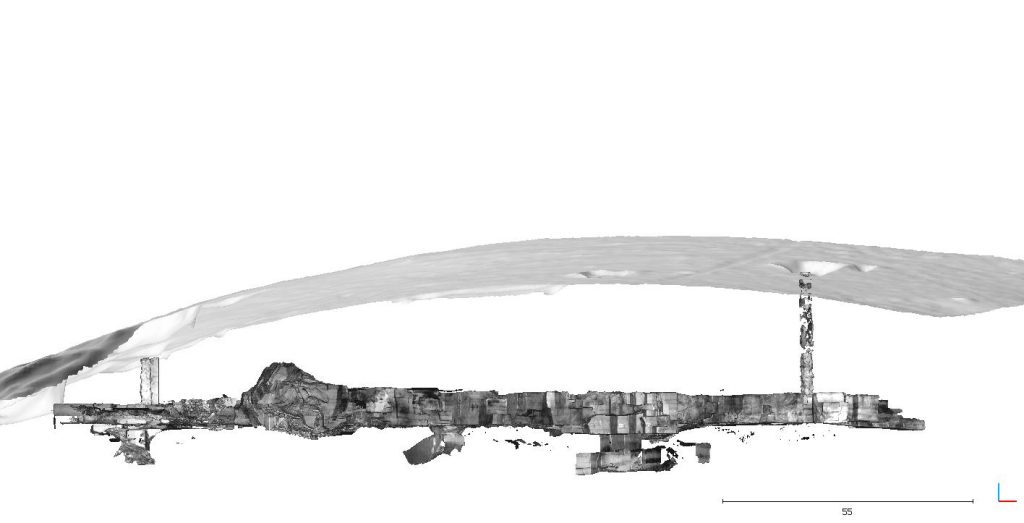
3D-Models
Impressions
References
Cloos, H. & Cloos, E. (1927): Die Quellkuppe des Drachenfels am Rhein. Ihre Tektonik und Bildungsweise, Zeitschrift für Vulkanologie, 11, 33-40
Frechen, J. (1976): Siebengebirge am Rhein, Laacher Vulkangebiet, Maargebiet der Westeifel: vulkanologisch-petrographische Exkursionen, Sammlung geologischer Führer (56), 3. Aufl.: 209 S.; Berlin: Borntraeger
Knaak, M & Strauß, R., Montanhistorische und geotechnische Untersuchungen in der Aero-Stahl-Grube. In: Zeugen der Landschaftsgeschichte im Siebengebirge – Teil 2: Der Ofenkaulberg. Kulturlandschaftspflege im Rheinland Bd. 4, LVR; 2020, S. 259 – 267.
Kolb, M., Paulick, H., Kirchenbaur, M., Münker, C. (2012): Petrogenesis of mafic to felsic lavas from the Oligocene Siebengebirge volcanic field (Germany): Implications for the origin of intracontinental volcanism in Central Europe. Journal of Petrology, 53, 2349-2379.
Leischner, W. (2006): Siebengebirge, das riesige Geotop am Rhein: eine erdgeschichtliche Dokumentation seiner Entstehung und Überformung sowie seinen Naturbausteinen und Lagerstätten; Gemeinschaftsausgabe vom Heimatverein Heisterbacherrott im Siebengebirge. 1. Aufl.: 76 S.; Siegburg: Rheinlandia-Verlag
Moll, M., Die Trachyttuffe im Gebiet Nachtigallental – Ofenkaule (Siebengebirge). – Bericht zur Diplomkartierung, 2006; Bonn.
Schwarz, C. (2014): Die Geschichte der geologischen Erforschung des Siebengebirges. 2. Aufl.: 194 S.; CreateSpace Independent Publishing Platform.
Zeugen der Landschaftsgeschichte im Siebengebirge (2020): Der Ofenkaulberg. – Landschaftsverband Rheinland (Hrsg.) 288 Seiten mit 357 Farb- und über 400 Schwarz-Weiß-Abbildungen